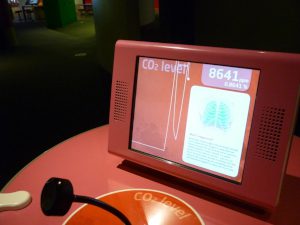
Researchers from MIT say they have developed a way of using carbon dioxide monitors to help estimate the risk of catching COVID-19 and other airborne diseases in near real time.
They say it could help track the evolving risk of transmission in indoor spaces such as schools and offices and may also lay the groundwork for the air quality monitoring systems of the future. Such systems could predict transmission rates of airborne diseases like COVID-19 or seasonal flu, and work in concert with ventilation systems to adjust the air within buildings to keep the risk of transmission low.
The research builds on work from the same authors, published earlier this year, which provided a guide to the risk of airborne transmission in different indoor settings. Using data from super-spreader events, they produced a mathematical model that estimated the average length of time it would take to become infected when sharing a space with someone who had COVID-19.
From this they produced a safety guideline, setting limits on time spent in shared spaces, and adjusted by factors including the size of room, numbers of infected and susceptible people, what they were doing and whether ventilation and masks were in use.
Writing in Flow: Applications of Fluid Mechanics, Martin Z. Bazant, professor of chemical engineering and applied mathematics, and John W. M. Bush, professor of applied mathematics, along with co-authors Ousmane Kodio, Alexander E. Cohen, Kasim Khan, and Zongyu Gu, explain that they have now been able to express the same safety guideline in terms of the concentration of carbon dioxide in the air – something that can be easily measured using simple, readily-available monitors.
The researchers say there is overwhelming evidence that the virus which causes COVID-19 is mainly spread by airborne droplets, breathed out by infected people. Measuring CO2 tracks how much air people are breathing out and the rate at which it is removed by ventilation.
The work of Bazant et al. combines those measurements with three models, which look at the dynamics of gas, of infectious aerosols – those virus-carrying droplets – and of disease transmission. They stress that concentrations of CO2 and of airborne pathogens are not strictly linked, as the amount of virus in the air is affected by a number of factors, including the use of face masks.
So their model takes into account other variables that include not only masks, but ventilation, the use of air filtration, activity levels and the number of people likely to be infectious or susceptible to infection at different stages of a pandemic. In all, more than 40 parameters feed into the model and produce an estimate of how much virus is present, and therefore the risk of infection, in near real-time.
This is expressed as an indoor reproductive number, which represents how readily the virus is transmitted to others, assuming a number of infectious people are in the room. This changes over time and is used by the researchers as a measure of safety: provided the number stays below a certain level, people can continue to share a space.
For simplicity, the model assumes that the main source of carbon dioxide in a room is people’s breath and that the air is ‘well-mixed’, meaning the concentration of CO2 is the same everywhere in the room. While there can be exceptions to this, such as when someone sneezes, the researchers say the assumption is supported by data from computer simulations and from real world events and is expected to be particularly good when the room’s occupants are masked.
These included two demonstrations they ran at MIT – one in a small office with two occupants, and the other in a lecture hall with 12 people. The CO2 concentrations were monitored, along with the number of people and whether they were talking or moving around.
The demonstrations showed it was possible to track a theoretical reproductive number and also highlighted the factors that increase or reduce danger. These included the impact of wearing masks, which dramatically increased the amount of time people could share a space without the reproductive number climbing above the safety limit. Filtration also increased the time taken to exceed safe limits, but by a much lesser extent.
The researchers acknowledge that the assumptions made and uncertainties about many of the parameters in the model, such as the concentration of virus in droplets of breath, and the relative susceptibility of different groups to infection, limit the accuracy of their guidelines. They hope these uncertainties will reduce as more data about real world spreading events is analysed.
Professor Bazant said: “CO2 monitoring has been used for decades to assess the quality of air handling in buildings, and can now be re-purposed to assess the risk of indoor airborne disease transmission, including COVID-19.
“We’ve shown how it can be used in conjunction with our safety guideline to assess that risk and hope to inform personal and policy decisions about closing and re-opening indoor spaces, such as schools and businesses.”
Together with app developer, Khan, the researchers have now made their model available as an online tool, to help people reduce risk to themselves and others in different indoor settings.