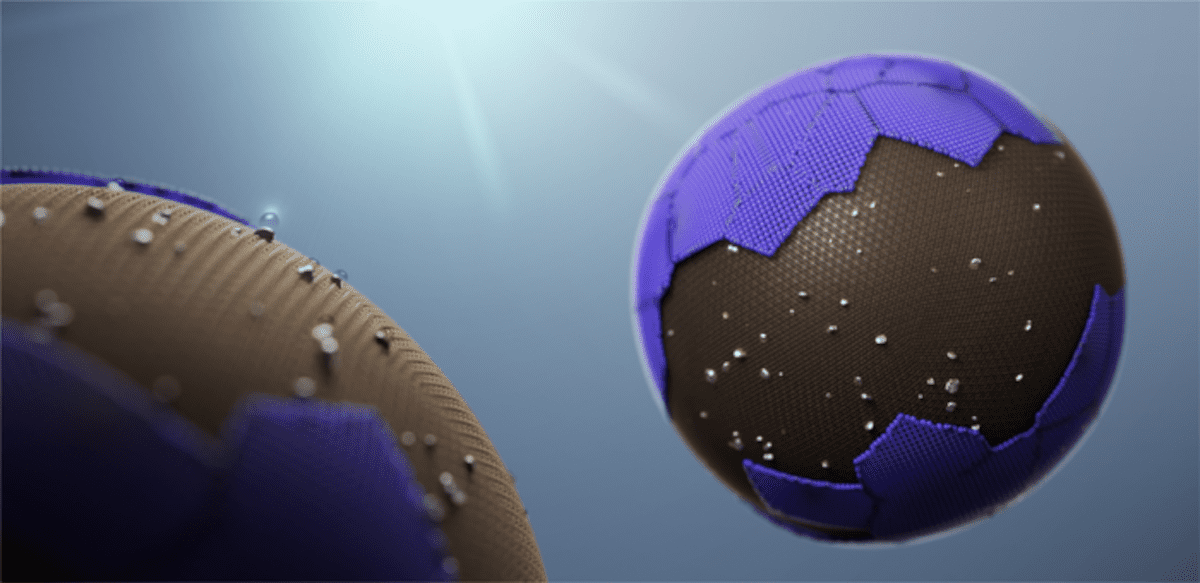
Sunlight is the most abundant source of renewable energy, but its inability to produce consistent energy levels over time means it struggles to satisfy the on-demand energy needs of much of the contemporary world. A promising option is to store solar energy as clean hydrogen fuel, derived from water by the so-called hydrogen evolution reaction (which occurs during the electrolysis of water) in the presence of a light-responsive catalyst.
Most hydrogen evolution photocatalysts consist of inorganic semiconductors, such as titanium dioxide, that almost exclusively absorb ultraviolet light. But because ultraviolet light represents less than five percent of the solar spectrum, the resulting photocatalysts are not efficient enough for commercial use.
An international team from KAUST, led by Iain McCulloch and postdoc Jan Kosco, were able to develop organic semiconductor-based photocatalysts by tuning the semiconductor bandgaps — which define the absorption wavelength range — to absorb visible light.
“All else being equal, the more light a photocatalyst absorbs, the more efficiently it can convert solar energy into hydrogen,” Kosco explains. “So, it is important to develop photocatalysts that are active over a broad range of ultraviolet-visible-infrared wavelengths to maximize light absorption.”
When exposed to light, semiconductor-based photocatalysts generate pairs of electrons and positively charged holes, or excitons, which dissociate into free charges that subsequently can migrate to the photocatalyst surface and drive hydrogen evolution. However, excitons are tightly bound in typical single-component organic semiconductors, which restricts charge separation and photocatalytic efficiency.
The researchers combined electron donor and acceptor semiconductor materials to form nanoparticles, known as heterojunction photocatalysts, whose overall bandgap configuration promotes exciton dissociation at the semiconductor interface.
“This is analogous to the bulk heterojunction used in organic solar cells,” Kosco says. “We, therefore, generated more charges in these nanoparticles than in those composed of individual semiconductors, which improved hydrogen production.”
Unexpectedly, the heterojunction resulted in extremely long-lived photogenerated charges in the nanoparticles.
“Charges typically recombine on the microsecond timescale, but we observed charges in our nanoparticles even a few seconds after photoexcitation, which is exceptionally long for photogenerated charges in organic semiconductors,” Kosco says. This is critical for catalyst performance because it gives more time for the charges to take part in relatively slow redox reactions at the nanoparticle surface, he adds.
The team is now exploring ways to apply the new photocatalysts to water splitting Z-schemes, where hydrogen and oxygen evolution photocatalysts are coupled to simultaneously drive the production of hydrogen and oxygen. They are also developing organic semiconductor photocatalysts for oxygen evolution.
The work has been published in Nature Energy.